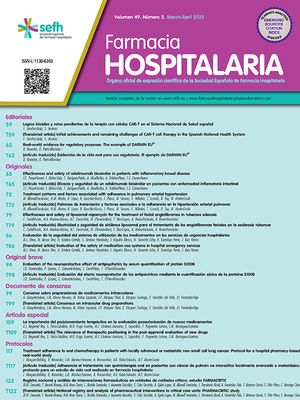
Nuevos roles y retos del farmacéutico de hospital. New roles and challenges of the hospital pharmacist
More infoThe aim of this article was to perform a narrative review of how pharmacogenetics and pharmacogenomics is being applied in the clinics, especially in Spain.
MethodPublications and websites of major interest have been reviewed.
ResultsPharmacogenes and variants used in several hospitals, available methodologies, and the implementation process are discussed.
El objetivo de este artículo ha sido realizar una revisión narrativa de cómo se está aplicando la farmacogenética y la farmacogenómica en la clínica, especialmente en España.
MétodoSe han revisado las publicaciones y sitios web de mayor interés.
ResultadosSe discuten los farmacogenes y variantes en uso en varios centros hospitalarios, las metodologías empleadas y el proceso de implementación.
Thanks to scientific and technological progress, identifying and applying the most effective therapeutic strategy for each patient appears to be within reach and remains the goal of most healthcare systems.1,2 Thus, the concept of precision medicine has emerged as the identification and application of the most effective therapeutic, diagnostic, and preventive strategy for each patient or population subgroup, taking into account genetic information and the influence of the environment.3 From the perspective of pharmaceutical practice, this concept involves the assessment of genomics, environmental exposure, lifestyle, and the analysis of other unique patient or disease characteristics in order to guide drug selection and dosing.4,5 In both cases, the focus is on genes or genomes, where genomes are understood as the combination of genes and other genetic elements rather than just the sum total of genes. This focus gives rise to pharmacogenetics and pharmacogenomics. Hereafter, we use the abbreviation PGx to refer to them interchangeably, as their objectives are essentially the same.
The implementation of PGx is expected to be hugely influential in modern society, where prescription drug use is very widespread.6 On the one hand, PGx implementation can help prevent adverse effects that require attention and, in many cases, hospitalisation; on the other hand, it can help select treatments and eliminate those that are ineffective. By genotyping the population, PGx can also be used to predict treatment.7 In fact, it is relatively common for individuals to possess genetic variants associated with PGx and to be exposed to multiple drugs over their lifetime, either serially or concurrently. Thus, PGx could help prevent adverse effects related to polypharmacotherapy.
Given this background, PGx implementation should be widespread; however, it appears that this is not the case. Therefore, we considered that it would be of interest to determine how PGx is applied in routine clinical practice in Spain (genes, variants, and methods) and the elements involved in its implementation.
MethodsSeveral resources were used to conduct this review: (1) The literature: A non-systematic review of the last 10 years of PubMed, WOS. The following terms were used as search terms: “Farmacogenética”, “Farmacogenómica”, “Medicina de Precisión”, “Medicina Personalizada”, “Farmacoterapia de Precisión”, and “Farmacoterapia Personalizada”, as well as their English translations: “Pharmacogenetics”, “Pharmacogenomics”, “Precision Medicine”, “Personalised Medicine”, “Precision Pharmacotherapy”, and “Personalised Pharamacotherapy”; (2) Databases: The Pharmacogenomics Knowledge Base (PharmGKB),8 Pharmacogene Variation (PharmVar),9 and ClinVar.10 PharmGKB contains information on pharmacogenes that have been approved by the US Food and Drug Administration (FDA),11,12 European Medicines Agency (EMA),13 Swiss Agency of Therapeutic Products (Swissmedic),14 the Japanese Pharmaceuticals and Medical Devices Agency (PMDA),15 and Health Canada (Santé Canada) (HCSC).16 PharmVar mainly contains CYP450 variants. ClinVar contains pharmacogenes and other biomarkers and is the reference for genotype–phenotype relationships in humans; (3) Clinical guidelines: From the American perspective, the Clinical Pharmacogenetics Implementation Consortium (CPIC)17 (cpicpgx.org), and from the European perspective, the Dutch Pharmacogenetics Working Group (DPWG)18–22; and (4) Spanish institutions and organisations: Consejo General de Colegios de Farmacéuticos (https://www.farmaceuticos.com/), Sociedad Española de Farmacia Hospitalaria (https://www.sefh.es/), Sociedad Española de Farmacogenética y Farmacogenómica (https://seff.es/), Sociedad Española de Farmacia Clínica, Familiar y Comunitaria (https://www.sefac.org/).
ResultsGenes, variants, and methodsThe pharmacogenes and methods employed are very similar in all the countries analysed. Table 1 shows the most commonly employed pharmacogenes. The table includes two Spanish examples because of the availability of detailed information. In total, 16% of the pharmacogenes analysed are transport proteins, 39% are metabolism proteins, and 45% are therapeutic targets. Thus, 55% of the pharmacogenes are bioavailability pharmacogenes (transport and metabolism) and 45% are therapeutic targets. The treatments involved show that the main therapeutic areas are antitumoral (23%), neurological (18%), immunosuppressant (11%), antiretroviral (10%), anticoagulant (8%), antihypercholesterolemic (8%), antidiabetic (8%), and biological (5%). These results are consistent with those depicted in Fig. 1 for services which offer PGx.
Pharmacogenes employed in projects and initiatives in Spain and other countries.
CPIC + DPWG | Sanford chip | PriME-PGx | La Paz | rsID (variants) | Protein family | Function | Treatments involved |
---|---|---|---|---|---|---|---|
ABCB1 | ABCB1 | rs2032582; rs1045642; rs3213619; rs1128503; | ATP Binding Cassette | T | Immunosuppressants and antiplatelet agents | ||
ABCC2 | rs717620; rs56296335; rs3740066; rs56199535; rs56220353; | ATP Binding Cassette | T | Antiretrovirals | |||
ABCG2 | ABCG2 | rs2231142; rs2273697; rs72552713; | ATP Binding Cassette | T | Statins, methotrexate, imatinib (tyrosine kinase inhibitor) | ||
APOE | rs7412 | Apolipoproteins (chaperones) | D | Anticoagulants, pravastatin | |||
CACNA1S | rs1800559; rs772226819; | Dihydropyridine receptor | D | Statins | |||
COMT | COMT | rs4680 | Catechol-O-methyltransferase | M | Catecholamines and derivatives | ||
CFTR | CFTR | rs75527207; rs113993960; rs199826652; rs267606723; rs193922525; rs80282562; rs121909013; rs74503330; rs12190909041; rs121908755; rs121909005; rs121908757; | Cystic fibrosis transmembrane conductance regulator | D | Ivacaftor | ||
CYP1A2 | rs2069514; rs762551; rs2470890; | Cytochrome P450 | M | Phenacetin, caffeine, clozapine, tacrine, propranolol | |||
CYP2A6 | rs28399433 | Cytochrome P450 | M | Letrozole, tegafur, coumarins, valproic, methoxyfluorane, disulfiram, halothane, fadrozol | |||
CYP2B6 | CYP2B6 | rs374527407; rs3211371; rs4803419; rs2279343; rs34223104; rs28399499 | Cytochrome P450 | M | Artemisinin, bupropion, cyclophosphamide, efavirenz, ketamine, methadone | ||
CYP2C19 | CYP2C19 | CYP2C19 | CYP2C19 | rs4244285; rs4986893; rs12248560; rs28399504; rs56337013; rs72552267; rs72558186; rs41291556; rs267606723; rs19393922525; rs80282562; rs121909013; rs74503330; rs12190909041; rs121908755; rs121909005; rs121908757; | Cytochrome P450 | M | Antifungals and antiplatelet agents |
CYP2C8 | CYP2C8 | Rs11572080; rs10509681; rs1058930; rs11572103; | Cytochrome P450 | M | Paclitaxel, psychotropics, oral antidiabetics | ||
CYP2C9 | CYP2C9 | CYP2C9 | CYP2C9 | rs1799853; rs1057910; | Cytochrome P450 | M | Psychotropics, anticonvulsants |
CYP2D6 | CYP2D6 | CYP2D6 | CYP2D6 | rs1080985; rs28371725; rs35742686; rs3892097; rs5030655; rs5030865; rs5030867; rs5030656; rs1065852; rs1058164; rs1135840; rs16947; rs28371706; rs61736512; rs769258 | Cytochrome P450 | M | Psychotropics, antinausea opioids (ondansetron) |
CYP3A4 | CYP3A4 | rs55785340; rs4646438; | Cytochrome P450 | M | Immunosuppressants | ||
CYP3A5 | CYP3A5 | CYP3A5 | CYP3A5 | rs776746; rs55965422; rs10264272; rs41303343; rs41279854 | Cytochrome P450 | M | Tacrolimus |
CYP4F2 | CYP4F2 | CYP4F2 | CYP4F2 | rs2108622; | Cytochrome P450 | M | Anticoagulants |
DPYD | DPYD | DPYD | DPYD | rs3918290; rs55886062; rs67376798; rs1801159; rs1801265; | Dihydropyrimidine dehydrogenase | M | Fluoropyrimidines (5FU) |
ERCC1 | ERCC1 | rs11615; rs3212986 | Endonuclease excision repair | D | Cisplatin | ||
EPHX1 | EPHX1 | rs1051740 | Endonuclease excision repair | M | Cisplatin | ||
FCGR2A | rs1801274; | Epoxide hydrolase | D | Immunotherapy | |||
G6PD | rs782669677 | Glucose-6-phosphate dehydrogenase | M | Primaquine | |||
HLA-A3101 | rs1061235 | Major histocompatibility complex | D | Carbamazepine, oxcarbazepine, lamotrigine, phenytoin | |||
HLA-B | rs144012689 | Major histocompatibility complex | D | Carbamazepine, oxcarbazepine, lamotrigine, phenytoin | |||
HCP5 | rs2395029 | Major histocompatibility complex | D | Retrovirus | |||
HTR2A | rs6311 | 5-hydroxytryptamine receptor | D | Psychotropics. Serotonin 5-HT2A antagonists | |||
IFLN3 | IFLN3 | rs4803217 | Interferon | D | Hepatitis C | ||
IFLN4 | rs469415590; | Interferon | D | Hepatitis C | |||
IL10 | rs1800896 rs1800872; rs1800871 | Cytokine | D | Anti-inflammatory immunotherapy | |||
IL23R | rs7517847; rs10489629; rs11465804; rs1343151 | Cytokine receptor | D | Biological therapy | |||
IL28B | rs12979860 | Cytokine | D | Interferon. Ribaviria | |||
KCNJ6 | rs2070995 | Potassium channel | D | Methadone | |||
MTHFR | MTHFR | rs1801133; rs4846051; rs1801131 | Methylene tetrahydrofolate reductase | M | Methotrexate | ||
NUDT15 | NUDT15 | rs116855232 | Nucleotide hydrolase | M | Thiopurines | ||
OPRM1 | rs1799971 | mu-opioid receptor | D | Antidepressants | |||
BY | rs1057868; rs2868177 | Cytochrome P450 | M | Immunosuppressants | |||
RARG | rs2229774 | Retinoic acid receptor gamma | D | Doxorubicin | |||
RYR1 | rs118192172 | Receptor ryanodine | D | Statins | |||
SLC15A2 | rs2293616; rs2257212; rs1143671; rs1143672 | H+/peptide transporter | T | Metformin | |||
SLC22A1 | SLC22A1 | rs72552763; rs55918055; rs36103319; rs34059508; rs628031; rs4646277; rs2282143; rs4646278; rs12208357 | Organic cation transporter | T | Tramadol, metformin | ||
SLC22A2 | rs316019; rs8177516; rs8177517; rs8177507; rs8177504 | Organic cation transporter | T | Fampridine, metformin | |||
SLC22A6 | rs11568626 | Organic cation transporter | T | Acyclovir, zidovudine, didanosine, zalcitabine, lamivudine, stavudine, trifluridine, cidofovir, adefovir, tenofovir | |||
SLC28A3 | rs7853758 | Nucleoside transporter | T | Thiopurines | |||
SLCO1B1 | SLCO1B1 | SLCO1B1 | SLCO1B1 | rs4149056; rs2306283; rs56101265; rs72559745; rs56061388; rs55901008; rs59502379; rs56199088; rs55737008; rs4149015 | Organic anion transporter | T | Statins, irinotecan, oral antidiabetics, oestrogens |
TBL1Y | rs768983 | Transducin beta | D | Biologic therapies | |||
TLR2 | rs4696480; rs11938228 | Toll Receptors | D | Immunotherapies | |||
TLR9 | rs352139 | Toll Receptors | D | Immunotherapies | |||
TNF | rs1800629 | Tumour necrosis factor | D | Immunotherapies | |||
TP53 | rs1042522 | Tumour suppressor | D | Cisplatin | |||
TPMT | TPMT | TPMT | TPMT | rs1800460; rs1800462; rs1142345; rs1800584 | Thiopurine methyltransferase | M | Thioguanines. 6-mercaptopurine and azathioprine |
UGT1A1 | UGT1A1 | rs887829; rs4148323; rs34993780; rs35350960; rs55750087; rs4124874 | UDP-glucuronosyl transferase | M | Irinotecan. Acetaminophen, carvedilol, etoposide, lamotrigine. Simvastatin | ||
UGT1A4 | rs2011425 | UDP-glucuronosyl transferase | M | Irinotecan, paracetamol, carvedilol, etoposide, lamotrigine. Simvastatin | |||
UGT2B7 | rs7438135 | UDP-glucuronosyl transferase | M | Morphine, mycophenolate | |||
UGT2B15 | rs1902023 | UDP-glucuronosyl transferase | M | Benzodiazepines | |||
VKORC1 | VKORC1 | VKORC1 | VKORC1 | rs9934438 | Vitamin K epoxide reductase | D | Anticoagulants. Warfarin and acenocoumarol |
XPC | XPC | rs2228001 | DNA repair protein | D | Cisplatin | ||
XRCC1 | XRCC1 | rs25487 | DNA repair protein | D | Cisplatin, PPAR inhibitors (olaparib, niraparib, rucaparib) |
CPIC, Clinical Pharmacogenetics Implementation Consortium17; D, Target; DPWG, Dutch Pharmacogenomic Working Group18–22; Sanford chip23; PriME-PGx, Hospital de la Princesa24; La Paz; Hospital de la Paz25; M, metabolism; rsID, reference SNP cluster identification number, variant identification number in the dbSNP26; T, transport.
Implementation of PGx by clinical area (Spain). Numbers represent the number of hospitals offering PGx services. Data obtained from the SEFH.27
The number of variants analysed per gene is relatively small, and in most cases is limited to one. The “rs” reference numbers are shown in Table 1, allowing access to the description of each variant in the dbSNP database26 (https://www.ncbi.nlm.nih.gov/snp).
The main analytical method used is PCR and, to a lesser extent, arrays (results not shown). In the analysis of arrays, ad hoc variant panels are mainly used in Spain, whereas commercially available fixed panels are in wide use in other countries. Next-Generation Sequencing (NGS) is rarely used, except in research settings. Two types of sequencing are used: Whole Exome Sequencing (WES) analyses around 1%–2% of the genome and is the most common method; and Wide Genome Sequencing (WGS) analyses the entire genome. A unique aspect of NGS is the number of reads or fragments into which the DNA is divided. These are sequenced simultaneously (bulk sequencing) and then sorted to obtain the sequence of the sample.28 The most commonly used NGS is Long-Read Sequencing (LRS) with reads of up to 45 000.
Implementation of pharmacogenetics and pharmacogenomicsThe implementation of PGx in hospital settings is widespread, whereas it remains limited in the community setting. This situation is similar in other countries. In Spanish hospitals, pharmacogenetics units have been set up to meet the demands of different clinical areas, especially oncohematology (Fig. 1).27 In community pharmacies, the implementation of PGx is at an early stage and is very uneven. Unlike the situation in hospitals, there appears to be a lack of infrastructure and testing is outsourced to specialised laboratories.
PGx is being developed within the framework of specific programs or projects. In other countries, development is at its most advanced in the United States, with initiatives such as the Cleveland Clinic's Personalised Medication Program, the CLIPMERGE PGx Program, the eMERGE-PGx initiative, IGNITE, INGENIOUS, the 1200 Patients Project, and PREDICT.29,30 In Europe, the Netherlands has gone furthest in developing PGx. In fact, this country was the first to publish clinical guidelines (by the DPWG) and develop systems in which prospective data on a set of key pharmacogenes are collected and included in patients' electronic medical records.31 In Spain, noteworthy programs include those conducted by the Instituto de Salud Carlos III,25 la Sociedad Española de Farmacogenética y Farmacogenómica,32 Hospital de la Princesa,24 and the MedeA Project.33
In the Spanish autonomous communities, PGx development remains uneven34 (see Fig. 2). An analysis of the key elements of implementation shows that the most important factor is the existence of government plans and strategies, followed by public–private collaboration and training. The existence of a critical mass of well-trained PGx specialists and infrastructure is much less influential.
Precision medicine in Spain and key elements in its development. Data obtained from the Roche report.34 1) Cantabria, 2) Castilla La Mancha, 3) Canary Islands, 4) Aragon, 5) La Rioja, 6) Principality of Asturias, 7) Balearic Islands, 8) Extremadura, 9) Community of Valencia, 10) Navarra, 11) Madrid, 12) Region of Murcia, 13) Castilla y León, 14) Galicia, 15) Basque Country, 16) Andalusia, and 17) Catalonia. PGx, pharmacogenetics/pharmacogenomics; PM, personalised medicine.
PGx has evolved from the creation of specific units in hospitals to solve specific clinical problems to the study of panels or mass sequencing of population groups in centralised laboratories at regional and national levels. In this sense, a noteworthy initiative is the Collaborative Spanish Variability Server (CSVS),35 which is a database that has collected genomes and exomes of individuals allowing us to determine the prevalence of pharmacogenomic variants in the Spanish population.
DiscussionThe number of pharmacogenes in use is relatively low compared to the number of genes considered useful or actionable pharmacogenes (284 genes associated with pharmacokinetics and 771 genes associated with pharmacodynamics).17–22 The number of variants analysed also shows a similar discrepancy, which seems to be due to 2 main factors: biological significance and methods.
Regarding biological significance, 2 pharmacogenetic categories can be distinguished: those affecting bioavailability and those affecting therapeutic targets. The biological significance of the first group is evident, as these pharmacogenes have specific roles in drug absorption, metabolism, distribution, and elimination, and are therefore typically included in most protocols. However, the proteins involved in bioavailability have broad substrate specificity and are applicable to multiple drugs, allowing the same panels to be used in a variety of clinical situations. It should be noted that many of the proteins involved are inducible; thus, if a drug is taken with an inducing agent (e.g., alcohol or another drug) adverse effects may occur as a result of variations in protein levels. Furthermore, it should be considered that both inducibility and substrate specificity can have relevant effects in polymedicated patients.
In contrast, the biological significance of the second group is less clear, so it is not surprising that many pharmacogenes are proposed, but few are selected. In principle, the therapeutic target should be the protein responsible for the problem being addressed. Nevertheless, in most cases, the cause of the problem is an imbalance in the biological system, involving multiple proteins and the effect of the environment on them. In all cases, we are dealing with multifactorial problems. An example of this situation is depicted in Fig. 3, showing the network of factors and interactions in the case of sickle cell anaemia. As can be seen, abnormal HbS, which is the main cause of the problem, can be affected by other modifying proteins, environmental influences, and the physiological response of the individual. In this case, all of these elements should be analysed, because they give rise to different clinical phenotypes that can be treated individually; however, in practice, they are usually excluded if the effect of any of these factors is modest. Another reason why some targets are not used is that, in many cases, they have been identified as statistical associations rather than functional ones, making it unclear how they are affected. Finally, the environment can have as much impact as genes on protein function; for example, proteins may behave differently under varying pH levels. In other words, genetic studies alone do not provide a definitive basis for therapeutic decision-making. A comprehensive assessment of biological systems can be provided by analysing the metabolome, which represents the outcomes of protein action and environmental effects. In this sense, it is becoming increasingly common to include metabolite analysis alongside genetic analysis.
Some possible variants are not always analysed, which poses some level of risk from a methodological point of view. However, it is reasonable to use arrays with a limited number of genomic variants and to reserve sequencing for special cases. The concept of special cases refers to rare variants and structural variants; arrays, however, neither detect them nor allow for haplotype phasing. Rare variants can have a greater impact on gene function and expression, exhibit greater population specificity, and play relevant roles in the genetics of complex diseases.36 Structural variants refer to deletions, duplications, insertions, inversions, and translocations, as well as complex rearrangements, all of which are very common in PGx. Regarding phasing, it should borne in mind that although the genome is often discussed as a singular entity, each person actually has a dual chromosomal endowment. The position of variants on one or the other chromosome can lead to differences in the response to drugs such as alpelisib.37
The predominant sequencing method is Whole Exome Sequencing (WES), which does not analyse introns. PGx has detected variants of interest in introns. On the other hand, Long Read Sequencing (LRS) is gaining momentum due to the advantage of longer read fragments, which contribute to a reduction in sequencing errors. These errors occur because DNA contains numerous similar sequences. Consequently, when using short reads, multiple alternatives can arise. In PGx, LRS is the preferred choice to study variants in complex genes such as CYP2D6 or HLA.
Implementation of pharmacogenetics/pharmacogenomicsPGx has been implemented in hospitals, but could also be implemented at all levels of the healthcare system. In fact, much of PGx is focused on outpatient drugs, such as antidiabetics, statins, and so on. Cavallari et al have outlined a set of specific elements that should be considered when establishing a PGx service.38 These elements include patient selection, analytical genotyping procedures, computer systems, staff training, and quality control. Thus, hospitals are the preferred location for PGx implementation due to their operational facilities. There is also a historical reason for this situation: the majority of the initiatives have emerged from research projects, most of which have been hospital-based. Further PGx development is likely to occur mainly in out-of-hospital settings, and the increasing use of Internet-based gene panels may contribute to this trend. In this regard, the FDA has approved direct-to-consumers (DTC) genetic test kits, which assess risk markers for cancer (BRCA 1 and 2) and other diseases (G6PDH). Many of these tests can be done without prescription, and so there is a clear need to inform people with community pharmacies playing a key role.
However, despite significant advances in knowledge, it is striking that the implementation of PGx has been so slow, both in Spain and in other countries.39 The reasons given for this situation include a certain level of scepticism, technical difficulties, the lack of specialists, and budget constraints.31,40 Critics highlight the lack of clarity concerning pharmacogenes. Furthermore, genotyping tests and registration processes often lack standardisation. In addition, recognition by drug agencies, which employ varying different criteria, is relevant to PGx implementation. In Spain, at least those medicines for which the Spanish Agency of Medicines and Medical Devices and the EMA include PGx analysis in their data sheets should be included. Regarding technical difficulties, the issues primarily stem from managing the results rather than from the analyses themselves.41 Thus, the analysis of a vast amount of data to provide a solution necessitates reliable mathematical algorithms. This aspect has significantly improved with the evolution of artificial intelligence and the substantial increase in computational capacity, thus enabling the rapid identification and application of solutions. However, this aspect remains a challenge because many such databases lack structure and interoperability, rendering their integration nearly impossible. The analytical aspect has transitioned from the early, almost handcrafted techniques to today's easily applicable automated methods. Finding a laboratory is not difficult and, currently, the SEFF is drawing up a map of laboratories in Spain. In other countries, the availability of genetic testing is very widespread and can be consulted in the Genetic Testing Registry database.42 The number of specialists is gradually increasing and costs are steadily decreasing.
The implementation of PGx has paralleled progress in precision medicine, both in Spain and in other countries. Government plans and strategies have significantly shaped the development of precision medicine in Spain. For example, the Spanish strategy for personalised medicine was launched in 2020. Governments are investing heavily, especially in infrastructures such as the Spanish IMPaCT program.43 Another key aspect is collaboration between the public and private sectors. In the biomedical field, such collaboration has stemmed from government programs and the keen interest of private companies, which have recognised this field as a good business opportunity. Consequently, this synergy has contributed to the remarkable growth of biotech companies in Spain (https://www.asebio.com/en). This aspect, among others, has led to an increase in the use of biotechnological treatments and the associated increase in costs, which contrasts with the expected reduction following the implementation of PGx. Some authors have suggested that this trend could lead to social inequalities by limiting access to medicines for people in lower socioeconomic groups.44
PGx training is of particular concern as a critical mass of well-trained PGx specialists is needed for its implementation to become widespread. As mentioned, some biomarkers have strong scientific and clinical support, whereas others are merely statistical associations that have been proven to be misleading and to have clinical implications. Discriminating between them requires well-trained specialists. In both pharmacy and medicine, undergraduate training in PGx is very scarce and postgraduate training does not include PGx as a speciality. However, courses do exist, some of which are free of charge, such as those offered by the COPHELA consortium.45 The current trend is to form multidisciplinary teams in which pharmacists, with their training in pharmacokinetics and pharmacodynamics, should play a key role. In relation to PGx, pharmacists' responsibilities include promoting the optimal use and timing of PGx tests, interpreting PGx test results, and educating healthcare professionals, patients, and the general public about the field of pharmacogenomics.46,47 Setting up such teams in hospitals is straightforward and improving with the creation of translational medicine units. Although this aspect can be challenging in community pharmacy, it is not insurmountable, thanks to information and communication technology.
The concept of precision medicine and PGx have evolved simultaneously.44 Initially, the concept was captured by the term personalised medicine (i.e., where each person receives the most effective treatment while avoiding adverse effects). Subsequently, the concept was referred to as precision medicine, which includes population subgroups as well as individuals, thus avoiding the possible misinterpretation of the term “personalised”. The concept, which was originally based on genetics, has also evolved to include other factors. Medical records increasingly contain PGx data along with so-called Medically Actionable Predisposition conditions.23 Finally, there is a trend toward preventive medicine where PGx focuses on characterising populations in anticipation of possible treatments.48 This is a global trend,49 and a good example is provided by the GENOTRIAL project at the Hospital de la Princesa (Madrid, Spain). This approach is part of a broader global strategy to collect all the biomarkers that show variation.50 This new approach has been welcomed with great interest by the pharmaceutical industry for population selection in clinical studies.
ConclusionsThe application of PGx data in routine clinical practice in Spain is similar to that in other countries, and has been advancing in parallel with precision medicine. Key elements for its development are translational research, governmental support, and specialist training.
FundingNone declared.
Author contributionsThe authors declare that they have contributed equally to the concept, design, definition of intellectual content, literature search, data collection and analysis, and the preparation, editing, and review of this manuscript.
Liability and assignment of rightsAll authors have fulfilled their responsibilities as defined by the International Committee of Medical Journal Editors (available at http://www.icmje.org/).
In the event of publication, all authors exclusively cede the rights of reproduction, distribution, translation, and public communication (by any means or sound, audiovisual, or electronic support) of this article to Farmacia Hospitalaria and by extension to the SEFH. To this end, a letter of ceding of rights will be signed at the time of sending the article through the online manuscript management system.